Introduction.
There is no “cure” for drug addiction, however there are drugs currently being used, or being developed, to help addicts cope and to help them quit. A comprehensive listing is beyond the scope of this post. Here, I focus more on the strategies than on the drugs themselves.
When employed, results are often mixed, working better for some addicts than others. Even when there is some effectiveness, compliance is often a problem. Since some of the treatment drugs are themselves addictive, some people are philosophically opposed to substituting one addictive drug for another. Some critics also argue that treatment drugs are just temporary “crutches.” Many treatment strategies are also complicated by addicts being addicted to more than one drug. Nonetheless for some individuals, these treatment drugs clearly improve the addict’s situation.
I am not a clinician so please consult a more comprehensive source or a clinical professional for information/advice about specific treatments. It’s very sad that around 40 to 60% of recovering addicts relapse within 1 year. At the end of this post, I provide links to resources which might be helpful.
Different ways that a drug can interact with a receptor.
There are variety of ways a therapeutic drug can interact with brain receptors to produce its effect. A drug can be a full agonist, partial agonist, neutral agonist (also called a receptor blocker) or an inverse agonist for the brain receptor that both it and the addictive drug utilizes. A full agonist is capable of causing a receptor system to produce its maximal response, with the others have progressively less capability, with the inverse agonist actually producing the opposite effect. All of these classes of drugs have been used in the treatment of drug addicts. Figure 1 illustrates the differences in receptor responsiveness to these different classes of drugs.

Full Agonist substitution.
Agonist substitution basically involves switching to a different, but equally efficacious, drug and/or to a different method of administration that is less harmful. The systematic use of this strategy began with heroin addicts substituting a synthetic opiate called methadone, another full opiate agonist. This strategy has since been applied to other drugs of abuse (e.g. nicotine gums for cigarettes, oral cannabis for smoked marijuana, amphetamines for cocaine). This strategy, when employed, attempts to achieve a number of goals.
One goal of this strategy is that the substitute drug should enter the brain more slowly so that it begins binding receptors more slowly while still satisfying the addict’s drug need. Slower initial binding causes less of a “rush” and less euphoria, allowing the addict to function more normally. This outcome can be accomplished in a variety of ways: by using a less lipophilic (fat-like) version of the drug which slows passage across the blood/brain barrier, by having the drug taken orally or by skin patch which causes slower entry into the blood and ultimately into the brain (versus intravenous, smoked, vaped, or snorted).
A second goal is that the substitute drug possesses a longer half-life to even out the addict’s drug response. For example, heroin’s relatively short half-life results in the addict’s entry into unpleasant withdrawal several times a day as the heroin begins to wear off. With the much longer half-life of methadone, these ups and downs are eliminated. In addition, a longer half-life also reduces the intensity of withdrawal should the addict miss a dose. Amphetamine (whose effects are virtually indistinguishable from cocaine) is sometimes substituted for cocaine, in part, for it’s longer half life. A longer period of effectiveness can also be achieved through timed release formulations.
A third goal is shifting to a safer method of administration. In the case of heroin users, that involves shifting from intravenous (I.V.) administration, which is fraught with disease hazard from dirty needles, to a much safer oral methadone administration. Oral amphetamine is similarly viewed as safer than administering cocaine by either I.V. administration, snorting or vaping. Nicotine gum or skin patch is similarly viewed as less harmful than smoking.
And finally, from an economic/societal perspective, providing addicts access to legal drugs (such as methadone) is substantially cheaper than incarcerating them for using illicit ones. While this approach can also be better for the addict’s wellbeing, it doesn’t necessarily turn the addict into well-functioning member of society. However, it can reduce the addicts’ use of illegal drugs as well as criminal behavior to support their habit.
Partial Agonist substitution.
This strategy involves using an alternative drug that binds to the same receptor as the addictive drug, but is only capable of producing a partial response. The addict’s drug need is hopefully satisfied while producing a much milder high. At the same time, should the subject relapse, the substitute also serves as a receptor blocker to keep the original drug from exerting its more powerful effect. Some examples of this strategy are buprenorphine (e.g. Subutex ) for opiate addicts and varencline (Chantix and Champix) for nicotine addicts (used in smoking cessation).
Receptor Blockers (Neutral Agonists).
The idea here is that if the drug is no longer rewarding, the user will not use it and (hopefully) eventually lose interest in taking it. One way of keeping a drug from being rewarding is to block its ability to bind to brain receptors.
A receptor blocker works by binding the same receptor site as the addictive drug but, unlike the addictive drug, produces no effect on its own. However, by binding the receptor site, ideally with higher affinity than the drug, the blocker prevents the addictive drug from binding the receptor. Probably the best-known examples are naloxone (e.g. Narcan ) and naltrexone (e.g. ReVia and Vivitrol) used to block the rewarding effects of heroin, fentanyl, and prescription opiates (and also can be lifesaving in treating opiate overdose). One downside to the regular use of receptor blockers is that it causes the blocked receptors to upregulate (i.e. increase in numbers). If the addict should discontinue taking the receptor blocker and perhaps a few days later begin taking the addictive drug again, the increased number of induced receptors greatly enhances the likelihood of overdose.
Inverse Agonists.
Inverse agonism can occur if a receptor system possesses some degree of spontaneous activity in the absence of agonist drug binding. The inverse agonist can then suppress the spontaneous activity to produce its negative effect. Therapeutic drugs with this capability would probably be used more for their receptor blocking properties than for their inverse agonism (which ideally would be of small magnitude).
Rimonabant (Acomplia, Zimulti), a cannabis CB-1-receptor inverse agonist, provides a cautionary tale. This drug could, of course, be used to block the rewarding effects of marijuana. However, rimonabant was introduced in Europe in 2006 as a diet pill (by blocking food reward) and had off label use as an aid in smoking cessation (by blocking nicotine reward). Shortly thereafter, in 2008, rimonabant had to be withdrawn from the European market (and also was not approved for use in the U.S.) because its use was associated with an increased incidence of psychiatric problems including depression and suicide.
Some now think endogenous cannabinoids working through CB-1 receptors may help many forms of reward turn on the dopamine reward circuitry accounting for rimonabant’s therapeutic uses described above. However, rimonabant’s side effects not only disqualify it as a therapeutic drug, they also provide serious concerns for other therapeutic strategies designed to suppress the general capacity to experience reward.
Aversion Therapy Drugs.
The idea here is that if the use of a particular drug is made aversive, the addict will be disinclined to use it. Disulfram (Antabuse), a drug developed to treat alcoholics, makes alcohol consumption aversive by blocking aldehyde dehydrogenase, the enzyme that eliminates aldehyde buildup following alcohol consumption. This drug normally has little effect on its own. However when the alcoholic takes a drink, the resulting toxicity causes flushing, nausea, vomiting and anxiety. Needless to say, disulfram compliance can be a problem.
Drug Vaccines.
Another strategy that might be available in the near future is using vaccines against specific drugs. The antibodies stimulated by the vaccine would attach to the drug preventing it from crossing the blood/brain barrier. Without access to the brain, the drug would not be able produce its rewarding effects. However, you have to “trick” the immune system to get it to produce the required antibodies.
The problem is that most neuroactive drugs (e.g. cocaine, heroin, nicotine, etc.) have to be very small lipid molecules in order to slip through the blood/brain barrier. However, their small size normally prevents detection by the immune system. To make these small drugs recognizable, the drug must first be modified and then attached to a much larger carrier protein. If done correctly, such a drug/protein complex can then be used to make a vaccine that will stimulate antibodies against the drug. Should a vaccinated addict take the drug, the drug antibodies can then attach to the drug and prevent it from exerting its effects. ( A video by the NIH describes the process in more detail.)
While the technique works in principle, the problem so far has been in getting the human immune system to produce sufficient antibodies, or sufficiently active antibodies, to provide meaningful protection. However, vaccine developers haven’t given up, and vaccines for many drugs of abuse are currently in development (e.g. cocaine, nicotine, methamphetamine, fentanyl, fentanyl analogs, heroin, and oxycodone). A downside is that vaccination works only for the drug you have been vaccinated against. Other drugs could still be abused for their rewarding value.
Detoxification and Rehabilitation.
The drug strategies mentioned are used both in easing the addict’s ongoing problems and in trying to quit. All treatment strategies are more likely to work if the addict is strongly committed to the treatment. However, as noted earlier, I am not a clinician, so for a broader overview of drug rehabilitation, I refer you to an excellent on-line document by the Substance Abuse and Mental Health Administration (SAMHSA) entitled “What is Substance Abuse: A Treatment Book for Families?”
People seeking treatment should certainly research the possibilities first. If you’re concerned about alcoholism treatment, I also recommend a relevant article published in the New York Times.
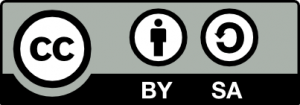