INTRODUCTION.

As seen in figure 1, we alternate between two different, and mutually exclusive, types of sleep: 1) Rapid Eye Movement (REM) sleep (red line) and 2) Non Rapid Eye Movement (NREM) sleep (black line, 3 stages). When we first fall asleep at night, we enter NREM sleep, the default sleep mode, accounting for around 75-80% of an adult’s sleep. However, NREM sleep is repeatedly replaced by REM sleep for short intervals (typically between 5 and 15 minutes). REM sleep accounts for around 20-25% of the night’s sleep.
The occurrence of REM sleep is controlled by a REM-Sleep Executive Center in the brainstem (hereafter referred to as the REM Center for brevity). The way I envision this working is that NREM sleep is the primary type of sleep, with REM sleep being highly modified NREM sleep, its various modifications orchestrated by the REM Center.
Whenever the REM Center becomes active, REM sleep abruptly appears. In order to cause the various characteristics of REM sleep, the REM Center oversees changes in a variety of other central nervous system locations. Other areas of the brain end REM sleep bouts by turning the REM Center off and also keeping the REM Center from turning on while awake. (Turning on while awake results in the narcoleptic sleep disorder known as cataplexy. More about that later.)
In an adult, the switching between NREM and REM sleep results in approximately 90-min (ultradian) sleep cycles, each cycle consisting of a period of NREM sleep followed by a period of REM sleep. We normally experience around 4 or 5 of these cycles each night.
The differences between the 2 types of sleep, as well as their mutual exclusiveness, is consistent with each serving different functions. However in most cases the proposed functions remain speculative. While a future post will address the functions of both types of sleep, this post lays some groundwork by examining the neuroanatomy and neurophysiology underlying REM Sleep and its “flip/flop” Control Switch. The various characteristics are described first and the post concludes with a more detailed (but still somewhat simplified) explanation of the workings of the REM Center.
CHARACTERISTICS OF REM SLEEP.
Although carried out in various brain and spinal cord locations, all REM sleep characteristics are initiated by the brainstem REM Center. In this section of the post, the various characteristics are described.
REM Center.
Figure 2 shows the approximate locations of the REM Center and the characteristics it controls. The REM Center is located in the reticular system of the dorsal pons and controls the REM sleep characteristics by sending out messages to the brain areas that cause these characteristics.

As in other posts, when describing brainstem neuroanatomy, a primitive vertebrate brain is used. A description of the primitive vertebrate brain, as well as the rationale for its use in describing neuroanatomy is covered in a previous post.
Workings of the REM Center.
The REM Center controls other brain areas by two processes (Figure 2). One process involves fiber tracts emerging from the ventral part of the REM Center utilizing glutamic acid (GA) as their neurotransmitter. Ascending GA neurons connect with forebrain areas, while descending GA neurons connect with the brainstem and spinal cord. The other process is an electrical disturbance called a PGO wave that propagates out from the REM Center to influence other brain areas.
Just to remind you, GA is the principle excitatory neurotransmitter in the brain and spinal cord, while gamma amino butyric acid (GABA) is the principle inhibitory neurotransmitter of the brain, and glycine is the principle inhibitory neurotransmitter of the spinal cord. All the other (100+) neurotransmitters function mainly to modulate the actions of these principle neurotransmitters.
It makes sense that GA neurons would be involved in controlling REM sleep. These large myelinated neurons allow messages to be sent quickly, often to CNS targets at some distance. In fact, throughout the brain, GA pathways and circuits are the primary basis for information transmission and processing.
A “flip/flop” switch mechanism (analogous to a light switch) insures that REM and NREM sleep are mutually exclusive. This switch arises from the reciprocal inhibition between the REM Center and another nearby brainstem area that I am calling the NREM Center. The NREM Center keeps the REM Center turned off both during NREM Sleep and during wakefulness (it should probably be called the NREM/Waking Center, but that’s too much typing!).
When experiencing NREM sleep or wakefulness, the REM Center is kept turned off by inhibitory input from the NREM Center (and other brain areas). However, at some point excitatory input into the REM Center overcomes this inhibition causing the REM Center to turn on, replacing NREM sleep with REM sleep. After some time (usually between 5 and 15 minutes), the NREM Center re-establishes control and sleep reverts to NREM sleep. This alternation continues over the course of the night with the abrupt transitions being very different from the gradualness of falling asleep or the gradual switching between different NREM sleep stages. More detail about all this later.
Although REM sleep is named for its rapid eye movements, other characteristics also distinguish it from NREM sleep. These include: bodily paralysis/atonia, cortical desynchronization, hippocampus and amygdala activation, and loss of body homeostasis.
ROLE OF GLUTAMIC ACID FIBER TRACTS IN REM SLEEP.
Glutamic acid (GA) fiber tracts originating in the REM Center control bodily paralysis, atonia, cortical desynchrony and hippocampal activity.
Bodily Paralysis And Atonia (loss of muscle tone).
The Central Nervous System controls the skeletal muscles with 𝜶- and 𝜸-motoneurons. The motoneuron cell bodies are located in the brain and spinal cord and their axons exit the CNS via cranial and spinal nerves to synapse on the skeletal muscles of the head and body. The 𝜶-motoneurons control muscle contractions by synapsing on extrafusal muscle fibers, while the 𝜸-motoneurons control muscle tone by synapsing on intrafusal fibers. When the REM Center is active, both types of motoneurons are deactivated, “disconnecting” these motoneurons from the muscles, producing muscular paralysis and atonia. Some motor activity does sometimes “sneak through” resulting in sporadic facial and limb twitches.
The reason it is important important to disconnect the CNS from the muscles during REM sleep is the individual is almost always dreaming. While dreaming, the motor cortex is generating output that would allow the dreamer to carry out the movements performed in his/her dream. The REM Center performs the important task of blocking this output and preventing sleepers from acting out their REM dreams. Acting out one’s dreams could potentially be dangerous since the dreamer is not aware of his/her environment.
Several lines of evidence support this protective function. For example, in cats, REM sleep paralysis can be selectively disabled (through several different procedures). Such cats appear normal when awake and fall asleep normally, but when entering REM sleep, they get up and begin acting out their dreams without respect to their environment (referred to as oneiric behavior). One interesting outcome is that we now know that cats dream about the typical things they do when awake: eating, drinking, meowing, purring, and hissing at, or attacking invisible objects! 🐈⬛
There is also a rare human sleep disorder called REM-Sleep Behavior Disorder (RBD) that parallels the oneiric behavior of cats. This disorder occurs most often in middle-aged to elderly males, although also seen in females and at younger ages. The episodes tend to occur in the latter part of the sleep period when REM dreams are the longest and most intense. The individual enters sleep normally but when an episode occurs, the person appears to wake up and begins acting out their dream. Multiple episodes per month are typical.
Since negative dream content is more common, the RBD individual may flail about, yell, and be at risk for accidentally hurting themselves or their sleeping partner. Around 80% of affected individuals later develop Parkinson’s Disease or related disorders caused by the buildup of 𝜶-synuclein, a brain waste product. RBD is different from sleep walking, which occurs only during NREM sleep. See a previous post for more detail on various sleep disorders.
The REM-Sleep Executive Center contributes to paralysis and atonia via 2 pathways (See Figure 3). In one pathway the REM Center sends messages via GA-releasing neurons directly to inhibitory interneurons in the medulla and spinal cord (shown here only in the spinal cord). The inhibitory interneurons synapse upon, and strongly hyperpolarize (i.e. inhibit) the 𝜶- and 𝜸-motoneurons. (Interneurons are small neurons with very short axons that influence other nearby neurons). This hyperpolarization renders the motoneurons incapable of producing action potentials, disconnecting the brain from the skeletal muscles. (The inhibitory interneurons of the medulla release GABA while those in the spinal cord release glycine.)
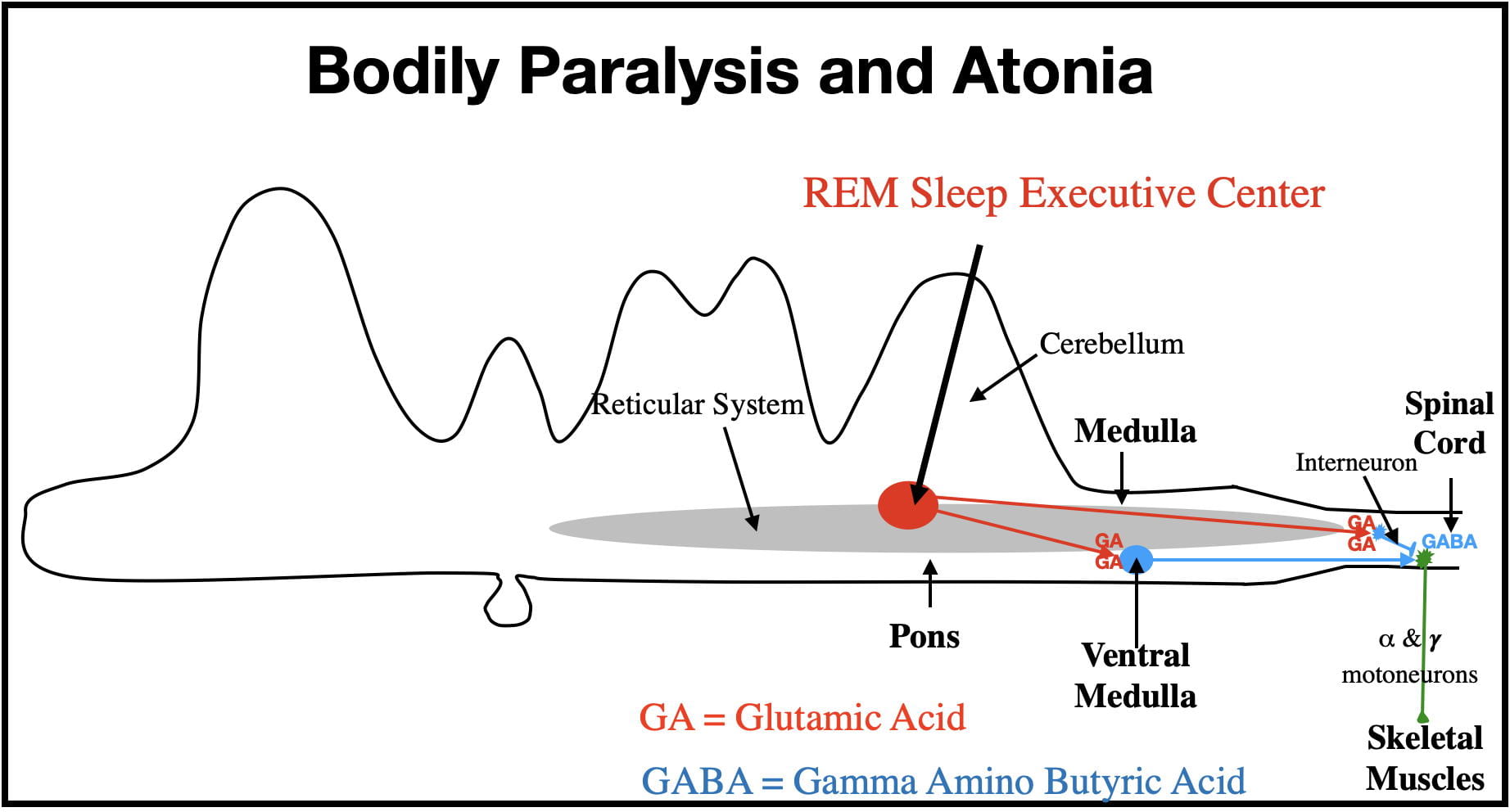
An alternate GA pathway involves the REM Center activating inhibitory GABA neurons in the ventral medulla. The longer axons of these neurons also synapse on, and inhibit, 𝜶- and 𝜸-motoneurons (see Figure 3). This pathway further serves to disconnect the brain from the skeletal muscles.
These 2 pathways represent an example of mother nature using redundancy to insure appropriate functioning in important biological processes.
Cortical EEG Desynchrony.
While passing through successively deeper stages of NREM sleep, the cortical EEG becomes increasingly synchronized (i.e. higher amplitude, lower frequency). However, during REM sleep, ascending GA fibers from the REM Center (figure 4) cause the cortical EEG to become desynchronized (lower amplitude, higher frequency), and resemble that of a relaxed awake, or lightly asleep, person.
As described elsewhere, a desynchronized EEG reflects the cerebral cortex employing its parallel processing capabilities. This processing involves sensory, motor and executive areas simultaneously working together to produce complicated mental processing. During REM sleep, this processing affects the qualities of our REM dreams! While dreaming can occur in NREM sleep, REM dreams are more complex, emotional, and intense than those of NREM sleep, reflecting the increased cortical processing.

Figure 4 illustrates the pathway by which the REM Center causes cortical desynchrony. Neurons of the REM Center release GA onto neurons in the Nucleus Basalis. The activated Nucleus Basalis neurons in turn project to the cortex where they release acetylcholine. Cortical acetylcholine elevations inhibit the cortical pacemaker neurons. As the pacemaker neuron influence diminishes, the sensory, motor and executive parts of the cortex all begin working together, resulting in the various qualities unique to REM dreaming.
Hippocampal Theta Waves.
The hippocampus is also activated during REM sleep by the REM Center’s ascending glutamic acid fibers. Like the adjacent cerebral cortex, the hippocampus is a “cortical” structure composed largely of grey matter (i.e neuron cell bodies). While the cerebral cortex is composed of neocortex (6 layers of neurons), the hippocampus is composed of simpler, more primitive archicortex (only 3 or 4 layers of neurons depending upon the classification scheme). However, like the cerebral cortex, the hippocampus can exhibit EEG brain waves. The theta waves during REM sleep reflect increased hippocampal activity.
One of the most important hippocampal “jobs” is to help the cortex consolidate short-term memories (reverberating electrical circuits) into long-term memories (structural changes in the cortex itself). These long-term memories are sometimes referred to as relational memories because the hippocampus helps not only in encoding these memories but also in relating (i.e “cross-indexing”) the memory to other cortical memories. The hippocampus also both “time stamps” and “place stamps” these memories, providing knowledge of the time and place that the memory occurred.

Relating a new memory to other memories makes the memory easier to recall by providing multiple paths for retrieval. Which makes it hard to understand why dreams are so difficult to remember. The difficulty appears related more to dysfunctional retrieval (bad crossindexing?) than memory storage. While the hippocampus does become more active during REM sleep, perhaps it is not as fully operational as when awake and alert.
Perhaps dreams are difficult to recall because their recall does not contribute to adaptation and fitness. As will be described in the next post, the neural processes that underlie REM sleep are thought to be functionally important. Perhaps the dreams themselves are just epiphenomena (i.e. going along for the ride). I’m sure many would disagree with the idea that dreams are unimportant. More about this in a future post.
As an aside, the memory problems of the early stages of Alzheimer’s Disease are caused mainly by the degeneration of hippocampal neurons (although cortical degeneration also contributes as the disease progresses). Hippocampal dysfunction has also been implicated as a cause of Major Depression and likely underlies the memory problems associated with depression as well.
ROLE OF PGO WAVES IN REM SLEEP.
As mentioned, some features of REM sleep are influenced by PGO waves. A brief explanation of PGO waves is presented first.
PGO Waves.
PGO waves are sporadic bursts of high voltage detected by macroelectrodes surgically implanted inside the brain. These high amplitude brain waves are caused by many nearby neurons firing their action potentials at the same time.
PGO waves were first detected in the pons, lateral geniculate nucleus, and occipital cortex of cats (giving rise to the PGO name), but can be detected in other brain areas as well. The waves originate in the Pons and then propagate out to the other brain areas. As PGO waves enter various brain areas, they contribute to REM-sleep responses. The waves resemble epileptiform waves, although not intense enough to stimulate seizures. Although PGO waves sometimes occur outside of REM sleep, they are highly symptomatic of REM sleep. However there are species differences. In mice and rats, for example, these waves are confined to the Pons and are called P-waves.
Bursts of PGO waves typically begin around 30 seconds before the onset of REM sleep. After REM sleep has begun, the waves recur intermittently as bursts of 3 to 10 waves. In between periods of activity are periods of quiescence. This relationship led to the idea that REM sleep is not a single state but is an alternation of two microstates: 1) a Phasic state when the PGO waves are active and 2) A Tonic state when they are not. The brain does appear to function differently in the two states. For example, during the Tonic state, the brain is more responsive to tactile and auditory stimulation (as measured by evoked potentials in the cortex) and the person is easier to wake up. However the overriding purpose of having 2 microstates is not clear.
Much of what we know about PGO waves has come from studying cats. Because the electrodes necessary to detect PGO must be surgically implanted, these waves have not been measured in healthy humans for obvious ethical reasons. However, PGO waves have been detected in a few “unhealthy” humans (with surgically implanted electrodes for either Parkinson’s Disease or Epilepsy). Consequently, it is safe to assume that PGO waves occur in healthy humans as well.
As the PGO waves travel to other areas of the brain, the waves influence local neural activity and functioning. For example, rapid eye movements as well as occasional facial and limb twitching correlate with the occurrence of PGO waves. The entry of the PGO waves into the occipital (i.e. visual) cortex likely also contributes to the strong visual component of human REM dreams.
Rapid Eye Movements.
REM sleep is named for its co-occurring rapid eye movements. While eye movements also occur during NREM sleep, these movements are less common, much slower, and the two eyes are uncoordinated. In the rapid eye movements of REM sleep, the two eyes move in concert.
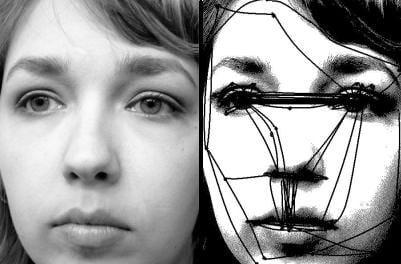
In fact, the rapid eye movements of REM sleep bear a strong resemblance to the highly coordinated saccadic eye movements of an awake person attending to, and scanning, some aspect of the visual field (See Figure 7). Saccadic eye movements are designed to allow us quickly to take in and analyze important aspects of our visual attention. They are also ballistic in the sense that once initiated, they are carried through to completion without midcourse corrections. And once completed, both eyes continue to have the same point of fixation.
The REM Center initiates PGO waves and the eye movements are eventually carried out by 3 cranial nerves controlling the muscles attached to the eyeball. What is not clear is the intervening neuroanatomy. There are 2 general hypotheses about what intervenes.
The leading hypothesis is that rapid eye movements are themselves saccadic eye movements related to the visual experiences of a dream. Motor cortex does attempt to carry out the body movements we perform in our dreams. However, as described earlier, this output is normally prevented from leaving the CNS. Perhaps rapid eye movements are similarly activated, but are not suppressed. Consistent with rapid eye movements being saccadic eye movements, PGO waves are also seen when awake cats perform saccadic eye movements.
While plausible, there are issues less supportive of this hypothesis. For example, the rapid eye movements of REM sleep are not quite as quick nor do they continue as long as the saccadic eye movements of an awake person. Rapid eye movements are also more likely to loop back to their starting point sooner than saccadic eye movements. Also, in contrast to expectations, individuals blind since birth exhibit rapid eye movements during REM sleep. And finally there are subcortical structures possessing the capability to influence eye movements independent of the cortex.
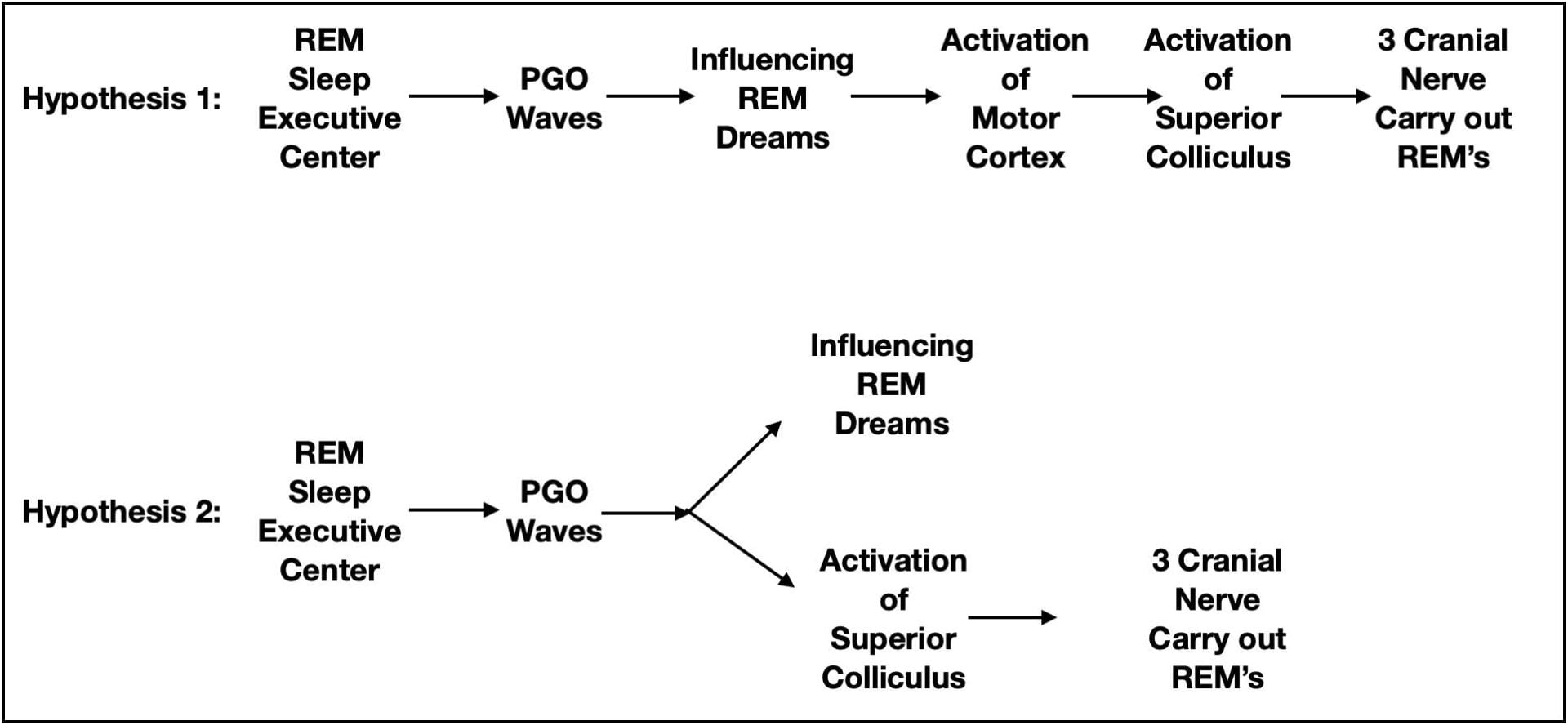
An alternate hypothesis is that the superior colliculus (an unconscious visual processing center in the midbrain), or perhaps even the motor nuclei of the cranial nerves controlling eye movements, are the intervening structures. If so, the rapid eye movements would be correlated with dreaming but not caused by dreaming (see figure 8).
More research is certainly necessary to understand the intervening neuroanatomy.
Amygdala Gamma Waves.
The amygdala is also activated by PGO waves. Like the hippocampus, the amygdala is part of the limbic system. One function of the amygdala is to assign emotional value to both stimulus input and behavioral performance. Also, like the hippocampus, the amygdala participates in the storage of cortical memories, but in this case, the storage of emotional memories.
Parts of the amygdala are composed of paleocortex (intermediate in complexity between neocortex and archicortex) and capable of exhibiting EEG brain waves. However, in the amygdala, highly desynchronized gamma waves, time locked to PGO wave bursts, reflect amygdala activation. The high levels of amygdala activity during REM sleep are thought to account for why REM dreams typically have a significant emotional component.
While the emotions in our dreams can be positive, negative emotions are more common.
Loss of Homeostasis.
There is also a loss of homeostasis during REM Sleep. Homeostatic processes exist to keep internal bodily conditions within acceptable limits. The unconscious autonomic nervous homeostatically regulates such bodily processes as breathing, heart rate, blood pressure and body temperature. These processes occur during both wakefulness and NREM sleep.
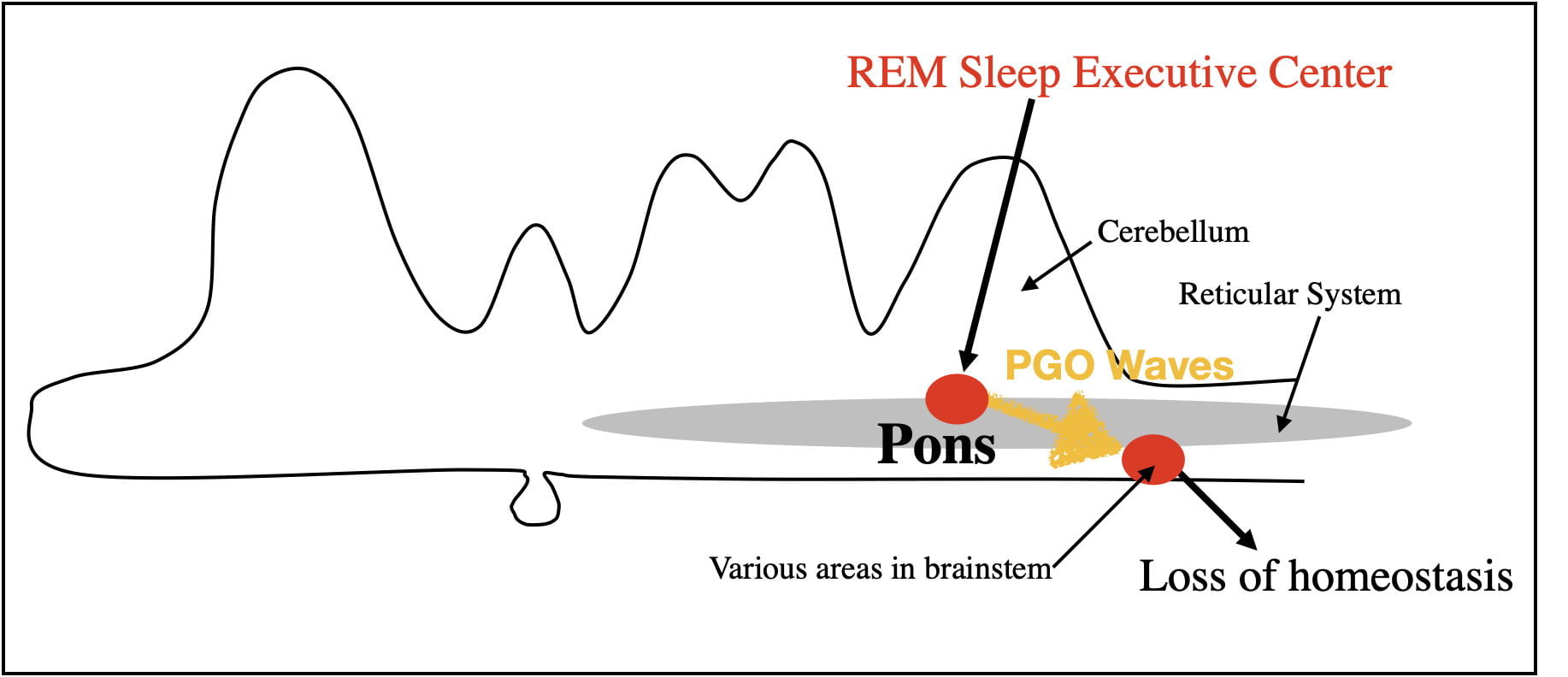
However, during REM sleep, homeostatic processes are disrupted by PGO waves. Breathing, heart rate, blood pressure and body temperature begin to fluctuate outside of normal limits. For example, the body is unable to respond to oxygen decreases by increased breathing rate, to decreases in external temperature by shivering, or to increases in body temperature by sweating. The loss of homeostasis also results in the nocturnal erections of the penis and clitoris independent of sexual arousal.
It is reasonable to assume that the evolutionary benefits of REM sleep exceed its costs. However, the loss of homeostasis during REM sleep is clearly a cost. Mother Nature appears to have minimized this cost by keeping REM bouts relatively short so as not to cause lasting consequences. As might be expected, small mammals, that are more likely to struggle to regulate body temperature, have shorter REM bouts. Also mitigating this cost, REM sleep is inhibited when sleeping in either colder or warmer temperatures than normal.
Perhaps the loss of homeostasis during REM sleep also accounts for why people prefer to sleep with a covering, even when it is not particularly cold.
NREM vs REM dreams.
If you define dreaming as mental activity during sleep, dreaming occurs in all stages of sleep. While the REM Center does not cause dreaming, it almost certainly influences the REM dream characteristics. As noted earlier REM and NREM dreams differ in a number of ways. While a sleeper is almost always dreaming during REM sleep, NREM dreams are more sporadic. The REM dreams also differ in having a more complex visual component, are more vivid, more bizarre, more emotional, and typically have a “story-line” in which the person is an active participant. These dream characteristics are clearly influenced by cortical desynchronization, PGO wave activation of the visual cortex, and amygdala activation.
Although NREM1 dreams can have a visual component, one researcher described these dreams as more like seeing a succession of photographs whereas REM dreams are more like viewing a movie. Perhaps some REM dreams spill over into NREM sleep where they become somewhat altered. There may also be differences between the different NREM stages. Mental activity during highly synchronized NREM3 sleep appears even less complex and more closely resembles the passive “thinking about things” when awake. These differences in REM and NREM dreams certainly beg future research.
NEURAL CIRCUITRY CONTROLLING REM SLEEP.
Keeping the REM-Sleep Executive Center Turned Off during NREM Sleep.
The model of REM sleep presented in Figure 10 represents my understanding of how REM sleep is controlled. The two structures of central importance are the REM Center and the NREM Center. The REM center consists of two interconnected nuclei with rather imposing names: the sublaterodorsal nucleus, and the caudal laterodorsal tegmental nucleus. This area is also sometimes referred to as the precoeruleus region. The NREM Center also consists of neurons from 2 regions: the ventrolateral periacqueductal grey and the lateral pontine tegmentum. Quite honestly, I have trouble keeping these anatomical names straight. However, it is not really necessary to remember these names to understand how the system works. The different inputs and outputs of the REM Center are numbered in figure 10 to allow the reader to follow along with the graphics.
As pointed out earlier, the REM Center controls the characteristics of REM sleep both by GA fiber tracts (9 and 10) and by PGO waves (8). During NREM sleep (and during wakefulness), the NREM Center inhibits the REM Center via GABA neurons (1). The NREM Center is also “helped” by two other inhibitory inputs (2 and 3). These 3 inputs are normally sufficient to keep the REM Center turned off during wakefulness and NREM sleep. The “flip/flop” switch mechanism that causes the abrupt transitions between REM and NREM sleep arises from the reciprocal inhibitory interactions between the 2 centers (1, 6 & 7). These interactions insure that when one center is “on”, the other is “off” and that switching between them is abrupt.
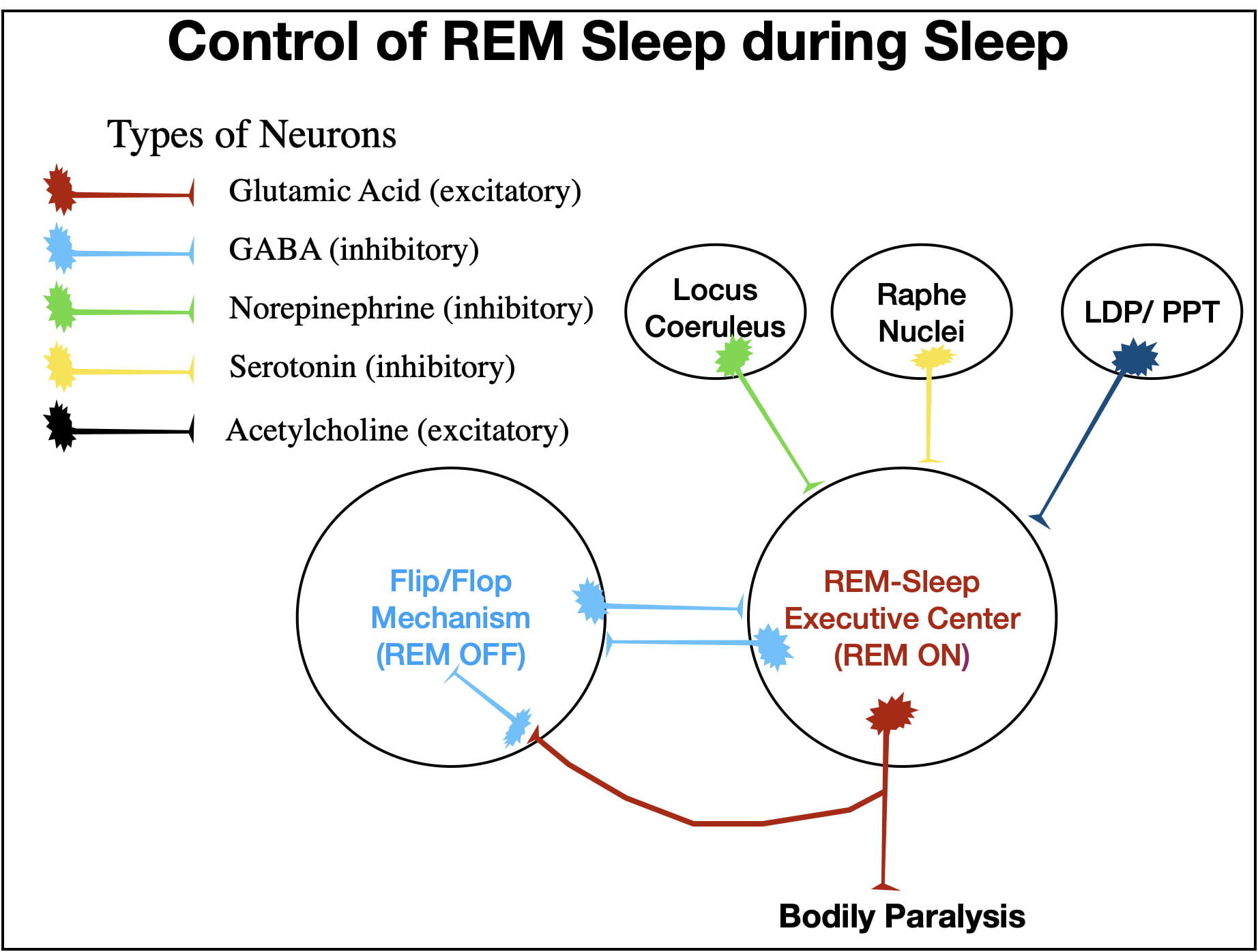
To understand how this all works, you need to know that the release of norepinephrine (2) and serotonin (3) into the REM Center follow a circadian rhythm. The greatest release of these neurotransmitters occurs when a person is awake and active, drop when awake and not active, drop even further through successively deeper stages of NREM sleep, and reach theirFigure 10. A Schematic illustrating some of the inputs and outputs from the REM and NREM Centers that are important in regulating REM sleep and in causing the abrupt transitions between REM and NREM sleep.lowest level during REM sleep.
During wakefulness the GABA inhibition from the NREM Center (1), coupled with that of norepinephrine (2) and serotonin (3), keeps the REM Center turned off. Although inhibition decreases during NREM sleep, it is still sufficient to keep the REM Center turned off.
However, just before a REM bout, neurons in the LDP/PPT (laterodorsal tegmental/pedunculopontine tegmental) areas release acetylcholine into the REM Center (4) which overcomes the inhibition (1, 2 & 3) and the REM Center turns on. An ascending GA fiber tract (10) brings about cortical desynchrony and hippocampal activation while a descending GA fiber tract (9) brings about body paralysis and atonia. In addition as PGO waves propagating out of the Pons (8) activate both rapid eye movements and the amygdala, and also cause the loss of homeostasis.
When the REM Center turns on, its GA-releasing neurons abruptly turn off the NREM Center via 2 pathways. Some GA neurons (5) activate inhibitory GABA neurons (6) that project from the REM Center to the NREM Center. In addition, GA neurons (7) also project to the NREM Center where they activate endogenous GABA neurons that also inhibit the NREM Center. (Yet another example of redundancy.) These two sources of inhibition (6 & 7) turn the NREM Center off.
After a variable period of time (usually between 5 and 15 minutes in humans), the NREM Center (1) along with the Locus Coeruleus (2) and the Raphe Nuclei (3) reestablish their inhibitory control and turn the REM center off. The person then abruptly switches back to NREM sleep.
Keeping the REM Center Turned Off During Wakefulness.
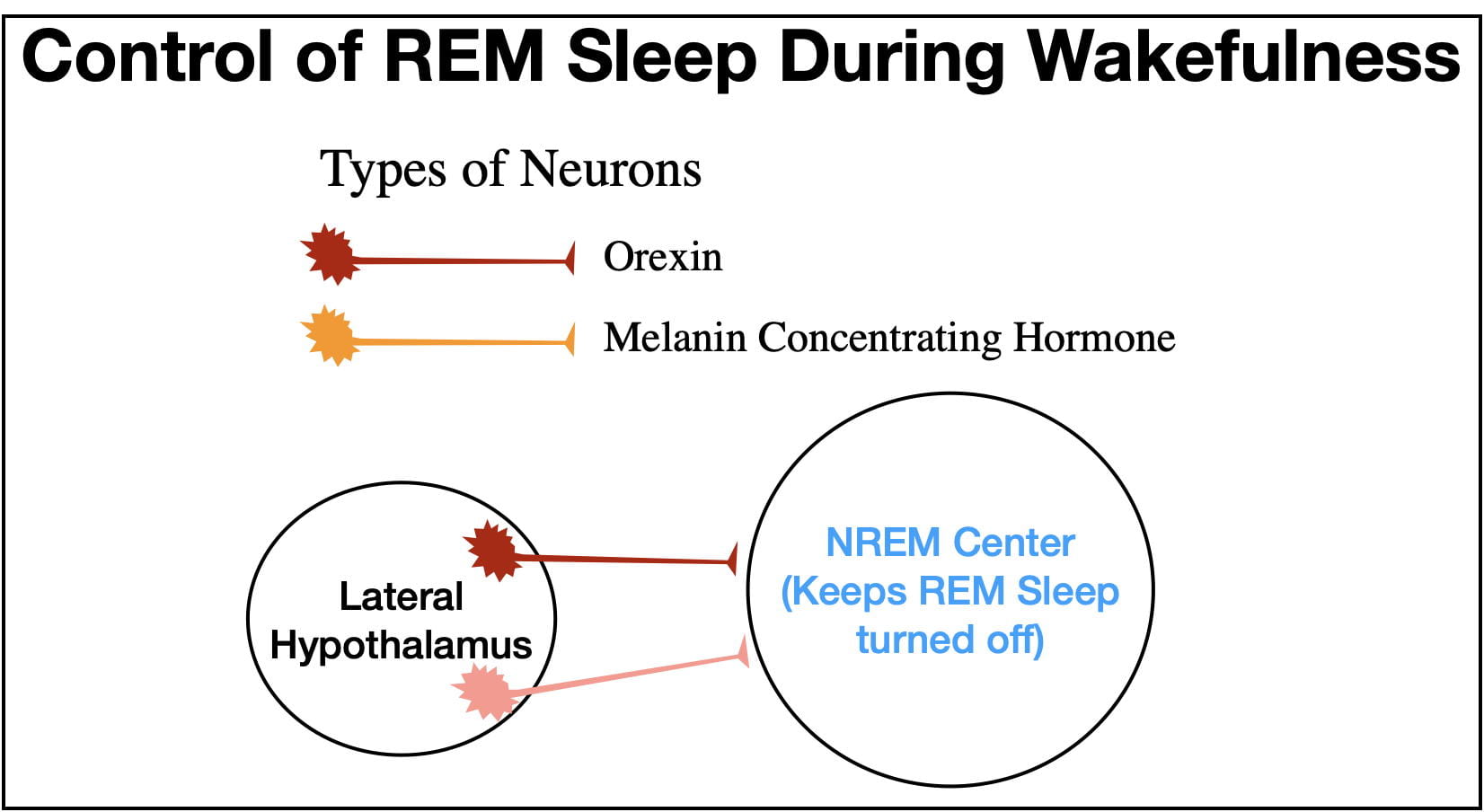
Two other neurotransmitters, Orexin (also called Hypocretin) and Melanin Concentrating Hormone serve the important role of insuring that the NREM Center keeps the REM Center turned off during wakefulness (see figure 11). Both neurotransmitters are released at their highest levels during wakefulness. (Melanin Concentrating Hormone was first discovered outside the brain where it serves as a hormone, but inside the brain it serves as a neurotransmitter).
However, it is possible for the REM Center to turn on during wakefulness. This is what happens in the narcoleptic sleep disorder known as cataplexy. In this disorder, emotion-provoking situations precipitate a cataplectic “attack” causing the person to collapse and remain paralyzed for the duration of the attack. Strangely, positive emotions, such as those that lead to laughing, are more likely to precipitate attacks than negative emotions. The person also sometimes experiences “dreaming” during the attack (called hypnopompic hallucinations) superimposed upon his/her waking consciousness.
The principle cause of cataplexy is the selective degeneration of orexin neurons. Without orexin input, the NREM Center is less able to suppress the REM Center, resulting in a cataplectic attack. Exactly why orexin neurons degenerate is not known, but cataplexy often runs in families reflecting a genetic predisposition.
CONCLUDING REMARKS.
When mother nature “figures out” a good way of accomplishing an outcome that benefits survival and fitness, that trait does not change much over evolutionary time. In some ways REM sleep fits this category. For example REM sleep occurs not only in terrestrial mammals, but features of REM sleep are seen in birds, reptiles and even some aquatic invertebrates such as cuttlefish (Peever and Fuller, 2017). Even some insects and spiders are thought to show features of REM sleep.
At the same time, substantial variation exists in REM sleep, even among mammals. As pointed out earlier “PGO waves” do not propagate out of the Pons in rats and mice as they do in cats and humans. Aquatic mammals like whales and porpoises apparently don’t engage in REM sleep at all while Northern Fur Seals engage in REM sleep only when sleeping on land. Perhaps in aquatic environments, where body heat can be lost quickly, the loss of homeostasis during REM sleep is too severe a “cost.” However, platypuses, another aquatic mammal, appear to have “solved” this problem by combining REM and NREM sleep into a single type of sleep. Despite these mammalian differences, the underlying neuroanatomy in different mammals is remarkably similar (Peever and Fuller, 2017) indicating that the REM neural circuitry itself is highly conserved!
The next post will examine the functions of sleep in general as well as those of REM and NREM sleep.
REFERENCES.
These references go into deeper detail on the neuroanatomy and descriptions of REM Sleep.
Corsi-Cabrera, M., Velasco, F., Del Rio-Portilla, Y., Armony, J. L., Trejo-Martinez, D., Guevara, M. A., & Velasco, A. L. (2016). Human amygdala activation during rapid eye movements of rapid eye movement sleep: An intracranial study. Journal of Sleep Research, 25(5), 576-582. doi:10.1111/jsr.12415 [doi]
Peever, J., & Fuller, P. M. (2017). The biology of REM sleep. Current Biology : CB, 27(22), R1237-R1248. doi:S0960-9822(17)31329-5 [pii]
Simor, P., van der Wijk, G., Nobili, L., & Peigneux, P. (2020). The microstructure of REM sleep: Why phasic and tonic? Sleep Medicine Reviews, 52, 101305. doi:S1087-0792(20)30048-4 [pii]
Wang, Y. Q., Liu, W. Y., Li, L., Qu, W. M., & Huang, Z. L. (2021). Neural circuitry underlying REM sleep: A review of the literature and current concepts. Progress in Neurobiology, 204, 102106. doi:S0301-0082(21)00120-9 [pii]
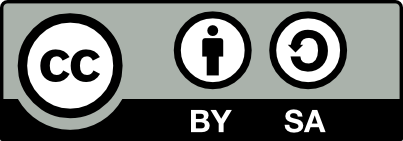