INTRODUCTION.
Sleep provides both next-day and long-term benefits to brain functioning. The next-day benefits include improved alertness, memory, problem solving, planning, decision making, mood, stress relief, balance, coordination, and sensory processing. The long-term benefits of sufficient sleep include a decreased likelihood of neurodegenerative disorders and a greater likelihood of living longer. This post begins addressing the underlying brain processes that give rise to these benefits.
For most of the body’s organs, sleep’s benefits occur because the body is resting. Although there is an overall 25% reduction in the brain’s metabolism during sleep, the brain does not appear to be resting. In fact, during REM sleep, the brain is often more electrically active than when awake. So if the brain is not resting, what is it doing to benefit itself? It is likely that multiple processes contribute to sleep’s benefits. However, it is surprising how little science knows for sure. While there are a number of hypotheses, most are educated guesses.
This post examines a recently discovered process, unique to sleep, that protects against the development of neurodegenerative disorders and likely also contributes to day-to-day brain functioning.
A PROBLEM (AND A SOLUTION?).
Despite being around 2% of a person’s body weight, the brain accounts for around 20% of the body’s metabolism. Because of its high metabolism, the brain produces a lot of metabolic waste. If not removed, the waste can become toxic and, over time, poison the brain’s neurons. The brain can take care of some of this waste through “recycling.” However the remainder needs to be removed. Fat-soluble waste capable of passing through the blood/brain barrier can be removed by the capillaries of the circulatory system. Some water-soluble waste can also be removed this way by virtue of being transported across the blood/brain barrier by selective transport mechanisms. However a substantial amount of waste can not cross the blood/brain barrier and must be removed by some other process. The lymphatic vessels of the immune system, which are capable of removing this waste, do not enter the brain. So, exactly how this waste might be removed was not understood until relatively recently.
In a series of 4 papers published in 2012/2013, Maiken Nedergaard proposed a process that operates primarily at night during the deepest stage of sleep that removes metabolic waste that can’t be removed by the circulatory system. Nedergaard named the process the glymphatic system because its waste-transporting vessels are produced by a type of glia cell unique to the brain (called an astrocyte) that function like lymphatic vessels outside the brain. Since 2012 over 1000 publications have examined different aspects of the glymphatic hypothesis.
A BRIEF ASIDE BEFORE PROCEEDING.
One of the things I learned from studying the philosophy of science many years ago is that while the scientific method can disprove a hypothesis, it can not prove one. All science can do is gather supportive evidence. When the totality of evidence is overwhelmingly supportive, the hypothesis is normally accepted (but not proven). While I am not an expert on the brain’s fluid dynamics, I find the evidence for the glymphatic hypothesis in a recent review (Bohr et al., 2022) pretty convincing. My bias in this post is to accept the glymphatic hypothesis. At the same time I note that there are scientists who interpret the findings differently (e.g. Hladky & Barrand, 2022). While I will briefly mention some of the disagreements, I will not be cover them in detail. But do keep in mind that even accepted hypotheses can later be rejected or modified in light of new evidence.
SOME BACKGROUND (BLOOD AND CEREBROSPINAL FLUID).
Since the glymphatic hypothesis depends upon movement of fluids and solutes among 4 interconnected fluid compartments, some background will be presented first. The 4 fluids that participate are: 1) the blood, 2) the cerebrospinal fluid (CSF), 3) The intracellular fluid of the brain’s cells, and 4) the interstitial (or extracellular) fluid between the brain’s cells.
Let’s begin with fluid movement between the blood and the CSF. The blood compartment is found within the arteries and veins while the ventricles and meninges house the CSF. As illustrated in the left side of figure 1, the ventricles consist of 4 interconnected cavities within the brain. Each cerebral hemisphere has its own lateral ventricle, while further down the brain, the third and fourth ventricles occur in the brain’s midline. The meninges, also seen in figure 1, are between the brain and skull and between the spinal cord and vertebrae.

While the blood and the CSF are normally prevented from exchanging fluid, there are two sites at which directional fluid exchange can occur: the choroid plexi of the ventricles and the arachnoid granulations of the meninges. The highly vascularized choroid plexi are embedded in the walls of each of the 4 ventricles and produce CSF by filtering blood, with the blood/brain barrier operative in this filtering. The resulting CSF is a clear watery liquid, similar to blood plasma (the liquid part of the blood without the red and white blood cells) but with much of the plasma’s protein and fatty acids also filtered out. As the CSF is produced, it is released into, and fills, the ventricles.
The continuous production of CSF by all 4 ventricles results in directional flow through the ventricles (as illustrated by the red arrows of figure 1). The CSF flows from the lateral ventricles to the third ventricle via a passageway called the Foramen of Munro. The CSF of the third ventricle flows to the fourth ventricle via a passageway called the Cerebral Aqueduct.
From the Fourth Ventricle, CSF flow continues into 2 additional structures. As seen in figure 1, CSF flows into and fills the Subarachnoid Space of the Meninges via passageways called the Foramen of Lushka and the Foramen of Magendie. In addition, CSF also flows into and fills the Central Canal of the Spinal Cord.
Because CSF is continuously produced, it must be continuously removed in order to maintain a constant volume of around 125 ml (4 oz). The Arachnoid Granulations embedded in the Arachnoid Layer of the Meninges perform the task of removal. The heavily vascularized Arachnoid Granulations resemble Choroid Plexi but perform the opposite task of moving fluid from the CSF back into the blood. There are also granulations at the lower end of the spinal cord that do the same.
The production, flow, and removal of CSF are very active processes. CSF has a half-life of only around 3 hours and is completely turned over around 4 to 5 times each day. If CSF is removed slower than produced, a condition called hydrocephalus (“water on the brain”) results. This condition occurs most often in newborns and the elderly. In this condition, hydrostatic pressure compresses the brain both from ventricles outward and from the meninges inward. If not promptly treated the compression can cause brain damage. In newborns, the compression can actually decrease the size of the brain and, because the skull is not yet ossified, enlarge the size of the head (a story for another day).
In addition to its role in glymphatic system functioning, CSF also performs other important functions. For example, CSF minimizes the occurrence of concussions by serving as a hydraulic cushion between the brain and the skull. The spinal cord is similarly protected. CSF also reduces pressure at the base of the brain. Since the brain is largely lipid and CSF is largely water, the brain “floats” in the meninges’ CSF, reducing its effective weight from around 1500 grams to around 50 grams.
There is little controversy about the fluid dynamics I have described so far.
THE BRAIN’S TOXIC WASTE.
While proteins are critical for brain functioning, they are also a major source of the brain’s water-soluble waste. A protein’s function is determined both by its amino acid sequence and how it folds into the appropriate 3-dimensional shape. Once synthesized and properly folded, proteins serve as receptors, membrane channels, membrane pumps, structural proteins, enzymes, neurotransmitters, and neurohormones. However, proteins are not forever. In fact, many brain proteins are functional for only a few days. When they cease to function, they become “waste” and must be gotten rid of (and replaced). If a dysfunctional protein, or its degradation products, are allowed to accumulate, either inside the neuron or in the brain’s interstitial fluid, they can aggregate into “plaques.” Over time, these plaques can become toxic and contribute to the development of neurodegenerative diseases.
In order to get rid of dysfunctional proteins, the cell is usually able to break them down into smaller units, typically a mix of peptides (protein fragments) and amino acids. The amino acids can be recycled to make new proteins (mother nature is a great recycler). However, the water-soluble, non-recyclable protein and peptide waste must be removed from the brain by some process other than by the circulatory system.
THE WORKINGS OF THE GLYMPHATIC SYSTEM.
My explanation of glymphatic functioning draws heavily from Bohr et al. (2022), a review cowritten by 19 scientists to share their understanding of the glymphatic system. This paper reviews what is known (and not known) about the glymphatic system and also proposes directions for future research and clinical application.
As seen in Figure 2, the first step involves the cellular waste being released by neurons (and glia) into the surrounding interstitial fluid. Once released, the glymphatic system can begin the process of removal. As mentioned earlier, the glymphatic system is largely inactive while awake and becomes functional primarily during the deepest stage of sleep (NREM3) that normally occurs during the first ⅓ of the night’s sleep. A 30-year-old typically gets around 2 hours of NREM3 sleep per night. On the other hand, septuagenarians like myself often experience as little as 30 minutes of NREM3 sleep each night.
As illustrated in figure 2, The proposed directional flow of fluid through the glymphatic system is illustrated by the large black arrows. The brain’s surface arteries initially run in the Pia Mater of the Meninges. Once the arteries leave the Pia Mater to penetrate the brain, astrocytes inside the brain send out projections called end feet that link together to form the arterial glymphatic vessels concentrically arranged around the arteries. Astrocytes also do the same around veins leaving the brain, forming the venous glymphatic vessels. The glymphatic vessels are also referred to as perivascular channels and perivascular spaces.
The unidirectional flow of fluid through the glymphatic system begins with the entry of CSF from the Subarachnoid Space into the arterial glymphatic vessels (see figure 2). Once in the arterial glymphatic vessel, CSF flow is directionally driven by pulsatile blood flow through the artery in the center of the glymphatic vessel. The fluid in the arterial glymphatic vessels, in turn, leaves these vessels by entering the interstitial fluid through a type of water channel called an aquaporin-4 channel. These one-way channels occur only in the outer walls of the glymphatic vessels and appear critical to glymphatic functioning. Genetic manipulations in mice that impair the performance of these water channels also impair waste removal while manipulations that enhance their functioning promote waste removal.
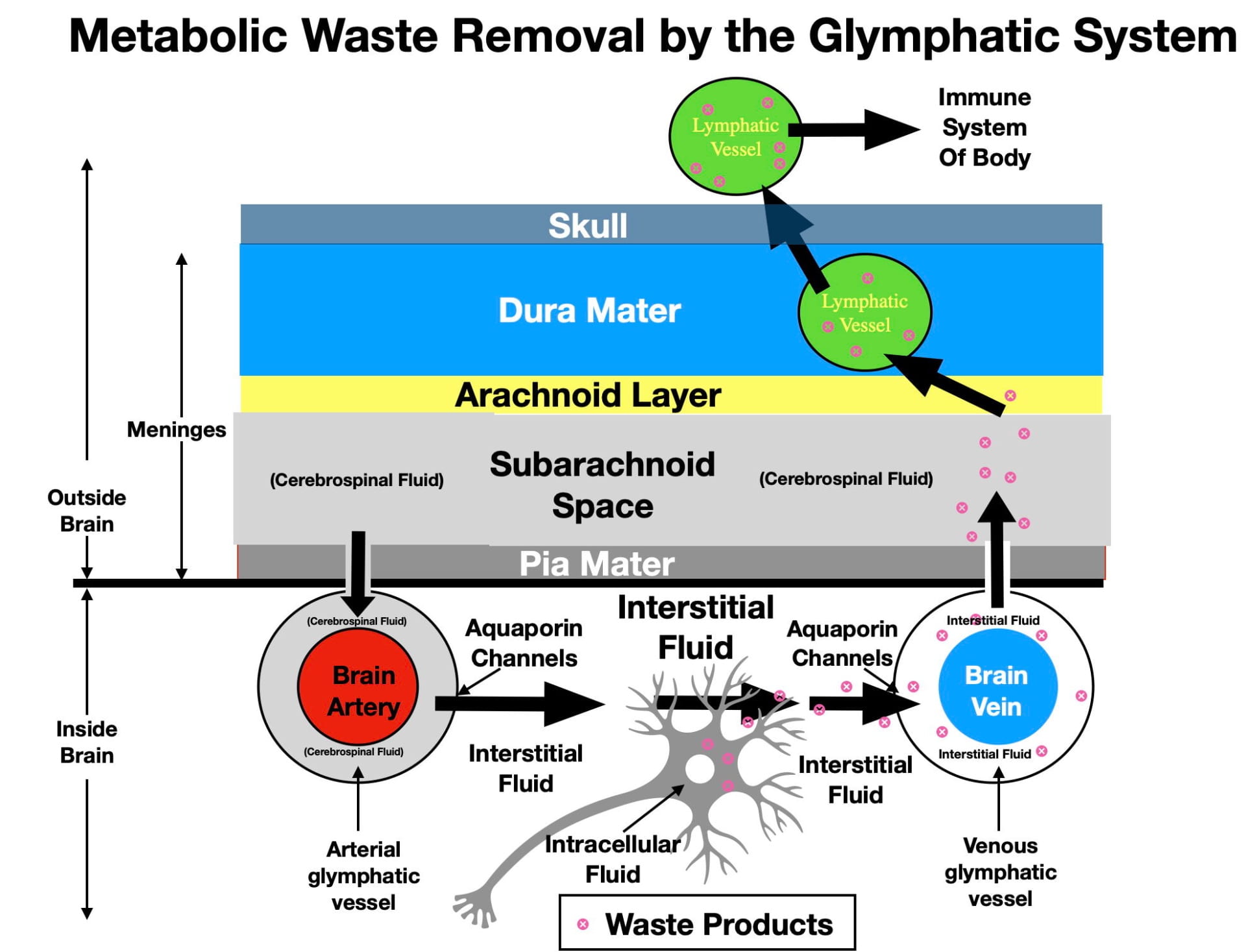
According to the glymphatic hypothesis, the interstitial fluid is thought to flow convectively from arterial glymphatic vessels to venous glymphatic vessels as illustrated by the black arrows of figure 2. In the process, the interstitial fluid picks up the metabolic waste released by the neurons and glia and transports it to the venous glymphatic vessels. The venous glymphatic vessels then absorb interstitial fluid with it’s metabolic waste, also aided by aquaporin-4 water channels. The directional flow in the venous glymphatic vessels is also thought to be driven by blood flow; however, in this case, the more even blood flow of the central veins. A Wikipedia animation of waste removal can be seen here.
The venous glymphatic vessels then release their waste-containing fluid back into the cerebrospinal fluid of the subarachnoid space of the meninges. There may also be efflux into the subarachnoid space by glymphatic vessels that run in the cranial nerves.
The last step involves waste removal from the subarachnoid space by the lymphatic vessels of the immune system. As mentioned earlier, the immune system does not enter the brain. However, in 2014, it was discovered that the immune system’s lymphatic vessels do participate in the brain’s water-soluble waste removal although they have a indirect way of doing so. Although lymphatic vessels don’t penetrate the brain, they do penetrate into the dura mater of the meninges. As seen in figure 2, this is close enough for lymphatic vessels to absorb waste from the subarachnoid space and transport it to lymph glands in the neck where it can be acted on by the immune system. Eventually the lymphatic system releases the waste into the circulatory system for excretion.
While awake, the glymphatic system is thought to be inefficient at waste removal because the small volume of interstitial fluid impedes interstitial fluid flow. Now comes the strangest aspect of glymphatic functioning (for me anyway)! During NREM3 sleep, the brain’s neurons and glia actually shrink in size by expelling some of their intracellular fluid into the interstitial fluid. As a result, the volume of the brain’s interstitial fluid expands by as much as 24%! This expansion is thought to facilitate interstitial fluid flow, enhancing waste removal. This expansion appears to be initiated by the delta EEG waves of NREM3 sleep. Anesthetic drugs that promote delta waves similarly increase interstitial fluid volume. In fact these drugs are now being considered as experimental therapies for elderly individuals with problems in experiencing NREM3 sleep.
(Hladky and Barrand (2022) argue that waste movement through the interstitial fluid is more by diffusion than fluid flow. Hladky and Barrand (2022) also argue that there is not good evidence for fluid flow in the venous glymphatic vessels and suggest that there could be efflux (as well as influx) via arterial glymphatic vessels and yet other mechanisms. The differences between Hladky and Barrand (2022) and Bohr et al (2022) are not that the water-soluble waste isn’t removed but rather in the possible methods by which it occurs.)
CONSEQUENCES OF BRAIN WASTE ACCUMULATION.
Regardless of exactly how waste removal is accomplished, if protein waste is not removed, it can poison neurons and, over time, cause neurodegenerative disorders. Which disorder develops is determined both by the type of waste that accumulates and the location in the brain where the accumulation occurs. For example, Alzheimer’s Disease is associated with the accumulation of two “waste” proteins, beta amyloid in neuronal cytoplasm and tau protein in the interstitial fluid of the forebrain. Parkinson’s disease is associated with the aggregation of alpha synuclein protein into Lewy Bodies in the cytoplasm of neurons in the midbrain, while Huntington’s, with fragments of a mutated form of the Huntington Protein in the cytoplasm of the forebrain. Other neurodegenerative disorders may be similarly caused.
The typical late age of onset of both Alzheimer’s and Parkinson’s Diseases is thought to be related to the diminished effectiveness of waste removal during aging. Huntington’s disease, which often has a younger age of onset, is caused by an inability to dispose of fragments of the genetically mutated Huntington Protein. While reduced glymphatic functioning often isn’t the central cause, it can permit genetic or environmental predispositions to better express themselves.
The brain has an incredible amount of redundancy built into its structure, so a significant number of neurons need to die before behavioral or cognitive symptoms become obvious. Consequently, the continued accumulation of waste for some time is usually necessary to precipitate obvious long-term symptoms of neurodegenerative disorders. At the same time, Bohr et al (2022) present evidence that loss of sleep for just one night has detectable effects on beta amyloid removal (a waste product associated with Alzheimers)! Consequently, I would suggest that even the less serious short-term consequences of a single sleepless night may be affected by glymphatic functioning as well.
Impaired waste removal with aging can also contribute to other types of pathology. A non-Alzheimer’s dementia called vascular contribution to cognitive impairment and dementia (VCID) appears enhanced by glymphatic system malfunctioning. In this disorder, atherosclerosis of the brain’s small arterioles not only impairs blood flow to the brain, it also depresses flow in the arterial glymphatic vessels which, in turn, depresses glymphatic functioning. Another medical problem in elderly surgical patients that may be related to reduced glymphatic functioning is the increased risk for delirium and impaired cognitive function during recovery from anesthesia.
OTHE BENEFICIAL CONTRIBUTIONS OF INTERSTITIAL FLUID FLOW.
The interstitial fluid flow of the glymphatic system also appears important for more than just removing waste! This flow is also thought to transport important molecules through the brain’s interstital fluid to appropriate brain locations where they are needed. These molecules include glucose, lipids, amino acids, neuromodulators, growth factors, cytokines, and neurotransmitters involved in volume transmission.
In addition, glymphatic flow is also thought to play a role in helping the brain monitor, and obtain feedback, from hormones and other substances in the blood. In order for this to occur, the substances must first enter the brain. However, protein and peptide hormones (such as insulin, glucagon, grehlin, leptin, etc) can enter only at “weak spots” in the blood/brain barrier. Once entering the brain (often near the hypothalamus), glymphatic interstitial flow is thought to deliver these substances to appropriate brain locations for detection and monitoring.
CONCLUDING REMARKS.
There is clearly more about the glymphatic system that needs to be discovered. Regardless of how the details turn out, the moral of this story is to regularly get a good night’s sleep, not only to function well the next day, but also to optimize your longevity and brain functioning into old age.
The next post will examine other beneficial brain processes thought to occur during sleep.
REFERENCES CONSULTED.
Bohr, T., Hjorth, P. G., Holst, S. C., Hrabetova, S., Kiviniemi, V., Lilius, T., . . . Nedergaard, M. (2022). The glymphatic system: Current understanding and modeling. iScience, 25(9), 104987. doi:10.1016/j.isci.2022.104987
Hladky, S. B., & Barrand, M. A. (2022). The glymphatic hypothesis: The theory and the evidence. Fluids and Barriers of the CNS, 19(1), 9-z. doi:10.1186/s12987-021-00282-z
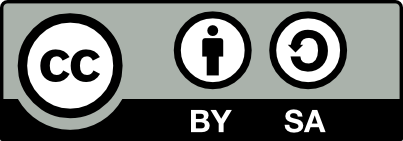